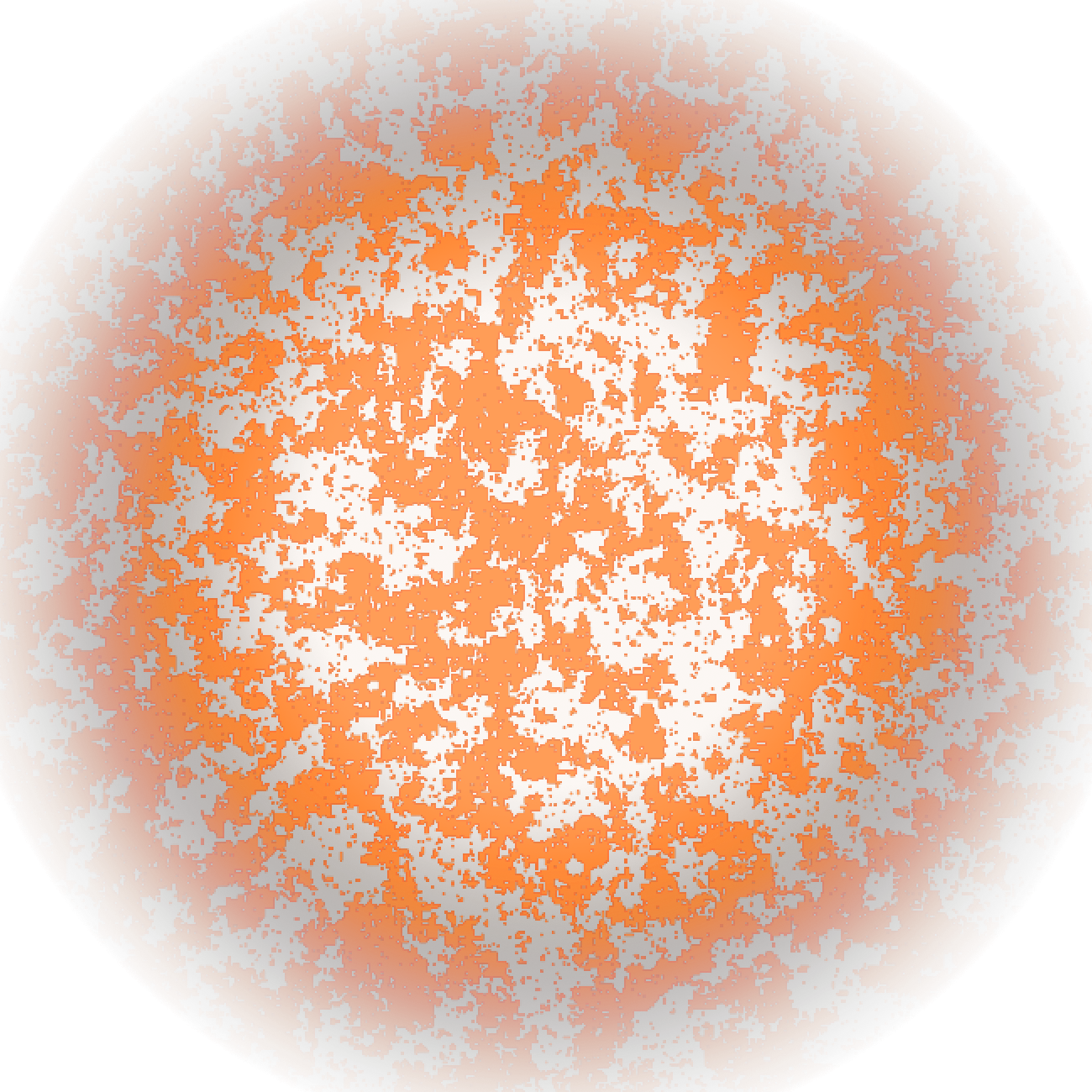
Econophysics
Many-body Dynamics of Production
In collaboration with economics professor Jesse Anttila-Hughes from USF, we apply techniques from statistical mechanics to study the emergence and growth of firms. This touches on fundamental questions such as how cooperation emerges and how physical limitations impact economic development. This field is rapidly growing and evolving, with an abundance of open questions and unexplored territory.
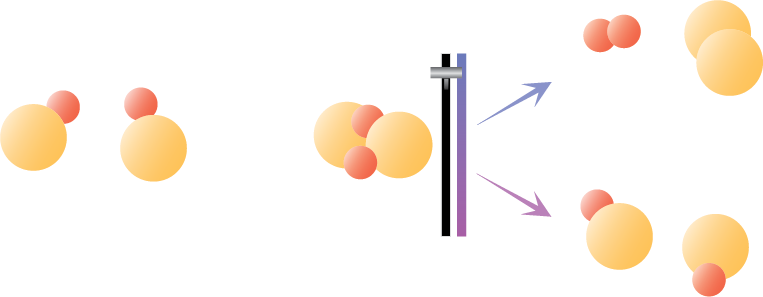
Quantum Chemistry
Ultracold and well-controlled
Chemistry is fundamentally quantum mechanical. The structures of molecules are determined by the quantum mechanics of strongly interacting electrons. To model even simple chemical reactions requires calculations of quantum dynamics too complex for modern computers. But, this underlying quantum nature is difficult to study in experiments because reactants can inhabit any of thousands of different states at room temperature. Ultracold chemistry opens a new realm.
At ultracold temperatures, we can completely control the quantum states of reactants. Instead of observing the average over a room-temperature mixture of quantum states, we can study reactions one state at a time. This gives us access to the quantum nature of chemical reactions. Learning about chemistry in this new regime holds promise to radically change our understanding of chemical processes. For instance, as the figure suggests, at these temperatures we can clearly explore means for controlling the outcome of chemical reactions with easily accessible experimental knobs.
A recent result from my previous group highlights this potential. Since brute-force calculations cannot adequately capture chemical dynamics, theorists attempt to build heuristic models of what behavior to expect in ultracold reactions. These models suggested that any chemical reaction not forbidden by a conservation law should be possible. In particular, when mixing triplet state NaLi molecules with Na atoms, a highly exothermic reaction was expected to occur. We found that for a particular choice of quantum states for the atoms and molecules, these reactions were suddenly turned off. While we continue to study the details of this effect, it immediately presented an application. We sympathetically cooled molecules with ultracold atoms, introducing a powerful tool to ultracold molecules research. You can get the details here: Collisional cooling of ultracold molecules.
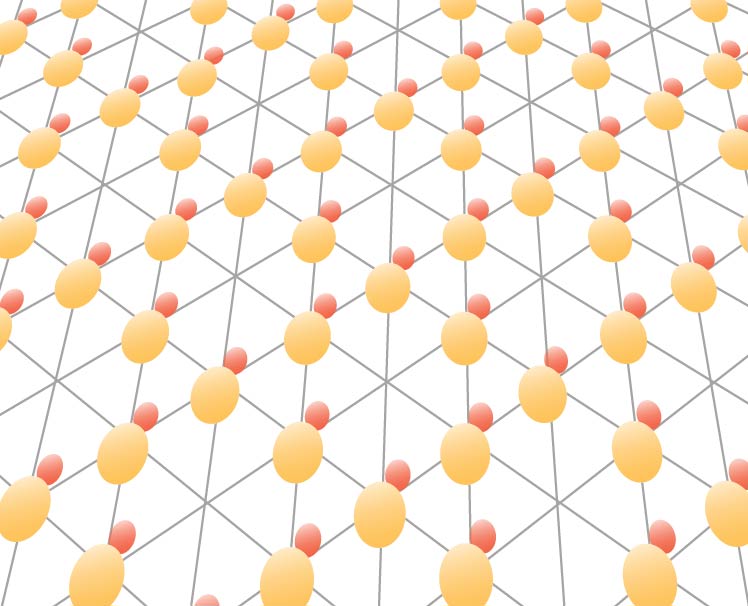
Quantum Simulation
Ultracold imitation games
Intriguing quantum many-body systems lie at the heart of many fields of research, from quark-gluon plasma in the early universe to high-temperature superconductors to the interior of a neutron star. Each of these systems is inaccessible to probes and controls at the single-particle level. The equations we believe describe these situations are usually too complicated to solve directly or with computers.
Ultracold atoms present a new possibility: quantum simulation. First, we pick the equations we believe describe our system of interest, for example the Hubbard model for high-temperature superconductors. Then, we find a way to apply lasers, microwaves, and magnetic fields to our atoms so that they will exactly obey the Hubbard model. Finally, we study the ultracold atom system, where we can control and detect with single-particle precision. This allows us to understand the Hubbard model and to see if its behavior matches that of the high-temperature superconductor. A nice example from my previous work was verifying the existence of a supersolid state for spin-orbit coupled bosons: A stripe phase with supersolid properties in spin–orbit-coupled Bose–Einstein condensates
Ultracold molecules have long-ranged interactions mediated by their permanent electric dipoles, which atoms lack. With ultracold molecules, we will have many new tools for building models of strongly correlated quantum systems that defy present understanding. The image depicts a configuration for studying frustrated spin models.
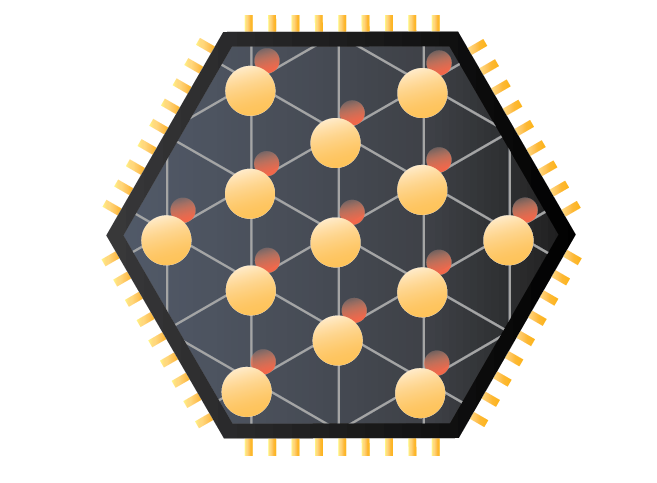
Quantum Computing
Putting the quantum to work
While strongly interacting quantum models cannot be solved by classical computers, quantum computers may be able to bring us new insight. A quantum computer uses the properties that make strongly interacting systems hard to calculate—entanglement and the large Hilbert space of a many-particle system—as resources for performing certain calculations dramatically faster than a classical computer. Those calculations can help us solve otherwise intractable models for quantum systems but they can also have practical applications in searching and cryptography.
Ultracold molecules are a promising system for quantum computing. At ultracold temperatures, molecules are extremely well controlled and can be isolated from external influences that could destroy the delicate states used in quantum computing. The long-range interactions that make molecules ideal for quantum simulation can also be used to perform the entangling gates necessary for quantum computation.